Cosmic Magnetism & Direct Observation of Reconnection-mediated Processes
Through decades of astrophysical research, we have established that magnetism is ubiquitous in our Universe, with interstellar gas, planets, stars and galaxies all showing the presence of magnetic fields. The challenge in studying cosmic magnetism is that, while stars and galaxies can be seen directly by the light they emit, magnetic fields are invisible even to the largest optical telescopes instead requiring the detection of polarised radiation, radiation which exhibits the effects of magnetic fields.
​
Recently, we caught one of the most illusive but ubiquitous magnetic processes that may be responsible for heating the Solar corona. Thanks to NASA's IRIS and SDO, we observed transient brightenings in cool, low-lying magnetic loops, which provided strong evidence for reconnection at sites of magnetic braiding in multi-stranded loops, leading to rapid, impulsive heating and the onset of transient brightenings. We reported profound evidence for preferential heating of abundant heavy ions and showed this is consistent with ion cyclotron turbulence due to the presence of strong currents at the reconnection sites.
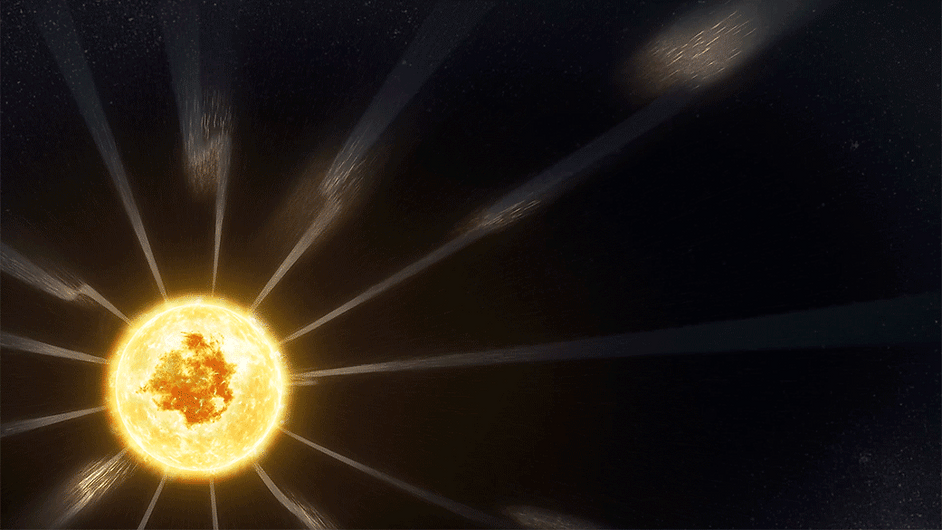
More recently, Parker Solar Probe showed us that close to the sun, solar wind—the outward stream of charged particles from the surface—is embedded with abrupt changes in magnetic field direction, called switchbacks, along which the solar wind flows at an accelerated speed. This novel phenomenon is possible tied with magnetic reconnection, known as interchange reconnection, but remains extremely difficult to model. We are developing multi-instrument techniques in collaboration with ISSI (Bern), UC Berkely, MSSL, and NSO to better diagnose this phenomenon and reveal the underlying physics.
Solar Photosphere observed by DKIST
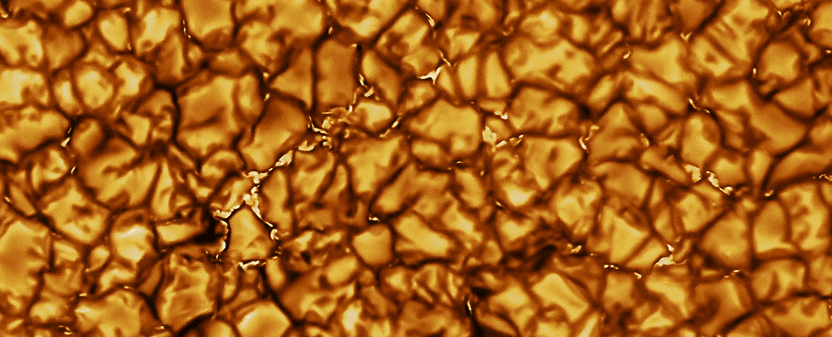
Listening Acoustic Wave Sources on the Sun
High resolution observations from the Imaging Magnetograph eXperiment (IMaX) revealed an abundance of transient, spatially compact large-amplitude Doppler velocity events on the solar photosphere. Detail statistics of such events exhibited strong agreement with the theory of superposition of strong convective flows and p-mode coherence patches. Since the Sun is a high-quality resonant chamber of plasma, these flows and perturbations reveal a great amount of information about its internal thermal structure. However, there remain significant “local” aspects of these motions that contribute to the evolution of the photosphere and the propagation of energy and momentum through it. Any investigation on the local sources of the photospheric perturbations requires a scheme that can reliably discriminate local perturbations from the background velocity (or pressure) field. Unfortunately, the very qualities that make the Sun an excellent resonant cavity also make it highly opaque to distinguish any individual local source: one can measure oscillations on the solar surface at a given time, but any measurement will be contaminated by contribution from many sources entangled in space and time. Inevitably an episode of recent emission from a local source becomes a miniscule component compared to the terrific accumulation of background noise constantly passing through the source region. This simple but stubborn problem has deterred scientists to probe local helioseismic sources and study their characteristics. In our group, we intend to hunt down these elusive sources and unveil the precise nature of the excitation events – the efficiency, phasing and relative importance of the source contributions, backed by direct observation.
XAI: Explainable AI to understand Solar/Stellar Dynamics
By the term stellar atmosphere we understand any medium connected physically to a star from which the light escapes to the surrounding space. In other words, it is a region where the radiation, observable by a distant observer, originates. Since in the vast majority of cases the radiation is the only information about a distant celestial object we have, all the information we gather about stars is derived from analysis of their radiation. Unlike laboratory physics, where one can change a setup of the experiment in order to examine various aspects of the studied structures separately, we do not have this luxury in astrophysics: we are stuck with the observed spectrum so we should better make a good use of it. In case of our Sun's atmosphere, we have powerful forward and inverse modeling codes that can treat high temperature plasma within a range of spatial and temporal scales. However, these codes are limited by our physical intuition and reasoning and thus they need constant revision as we make new observations and experimentation. Moreover, the more complexity we add to these models, the more heavier they become computationally. Hence, our group places observation driven modeling powered by Artificial Intelligence on the table. We collaborate groups of computer scientists in order to develop novel algorithms that are specifically catered for our needs. More importantly, our collaborator and we are building Explainable Deep Learning models that can facilitate knowledge extraction directly from observation, without the help of any underlying model assumption.
